3.7.3 Reactions at the Carbonyl Carbon
Learning Objectives
By the end of this section, you will be able to:
- Describe how carbonyl carbons participate in substitution reactions
Carbonyl compounds containing the polarized carbon-to-oxygen double bond exhibit several types of characteristic reactions. Some of these involve new bonds forming at the carbonyl carbon itself, with possible consequences for the carbon-oxygen bond. But a new bond to the carbonyl carbon can also initiate the disconnection of another group already attached there, resulting in the substitution of the new group for the leaving group.
There are lots of possible variations on these reactions we could consider. But to avoid overwhelming detail we will focus on a single example of this second type of reaction.
The reactants in this example include an ester (3-methylbutyl ethanoate) and an alcohol (methanol). The products of the reaction are a different ester (methyl ethanoate) and a different alcohol (3-methyl-1-butanol). In the course of the reaction the methoxy group from the reacting alcohol has been substituted for a portion of the original ester.

Note that portions of the original ester now exist in two separate molecules, and the methoxy group from the reacting alcohol shown in black is now incorporated into the product ester.
This substitution reaction is called a transesterification, indicating that the process starts with one ester and produces a different one.
A mechanism for this reaction might involve an attack by the oxygen from the methanol at the carbonyl carbon. Considering that the oxygen atom on methanol carries a partial-negative charge, it is reasonable to imagine it would be attracted to the electron deficient, partially-positive carbonyl carbon. Once there, the bond between those atoms can begin to form, but the carbonyl does not have the capacity to form another bond. As a result, the blue 3-methyl-butoxy group disconnects as the new bond forms.
Substitution reactions of a variety of types occur widely in nature and in the laboratory. Substitution at a carbonyl carbon is common in biochemistry, with variations on this mechanism useful for explaining many important biological reactions: the phosphorylation and dephosphorylation of ATP involved in energy metabolism, the formation of amide bonds in protein formation, and the catalytic activity of important digestive enzymes all fall into this category.
Making Soap: The Saponification Reaction
Saponification is a chemical process that involves the hydrolysis (breakdown by water) of fats and oils into their component molecules, glycerol and fatty acids. This reaction typically occurs in the presence of an alkali, such as sodium hydroxide (NaOH) or potassium hydroxide (KOH). The result of saponification is the formation of soap.
Soaps consist of a polar “head” and a nonpolar “tail.” The polar head is hydrophilic (attracted to water), while the nonpolar tail is hydrophobic (repelled by water). This dual nature of soap molecules makes them excellent at emulsifying oils and dirt, allowing them to be washed away by water.
View the following video to learn about saponification reaction.
Saponification reactions are similar to transesterifications. In this case the reactant attacking an ester is not an alcohol, however, but a hydroxy anion (OH-) from a strong base, NaOH for instance (sodium hydroxide/caustic soda).
The reaction generally involves oils (triglycerides) bearing ester groups, which react with a strong base (NaOH, caustic soda). The products of the reaction are glycerol (a polyalcohol) and the soap molecule (a fatty acid).

A reasonable mechanism for this process includes steps that are very similar to what we have considered above.

The mechanism includes an attack at the carbonyl carbon by the hydroxyl anion, and subsequently the disconnection of a group from the original ester.
The result of this reaction is the formation of a carboxylic acid anion (the form of the carboxylic acid after it has lost its acidic hydrogen) and an alcohol.
Reactions of this type are called saponification, and include the chemistry that is used to convert fats and oils to soap:
This chemistry is used in modern industrial soap-making processes but also describes the chemistry carried out on a small scale, as cottage industry or in the home. Triglycerides are the main type of fat storage in both plants and animals, and are the predominant material in both animal tallow (rendered fat) and plant oils. Reacting these substances with lye (NaOH) solution produces fatty acids. These substances act as soap, forming micellar structures as shown below. Micelles have hydrophobic, non-polar interiors that mix with and thus dissolve non-polar substances they contact, such as fats. The outer portion of the micelle is soluble in water and is hydrophilic, however, due to the oxygens and charge, the entire micelle, with fatty cargo in tow, can be removed from surfaces and washed away in a soapy water solution.
In this way soap is able to remove non-polar contaminants from dishes, fabric, and surfaces, including our bodies.
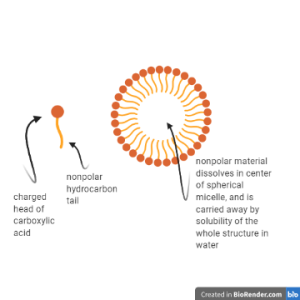