Anatomy of the human eye
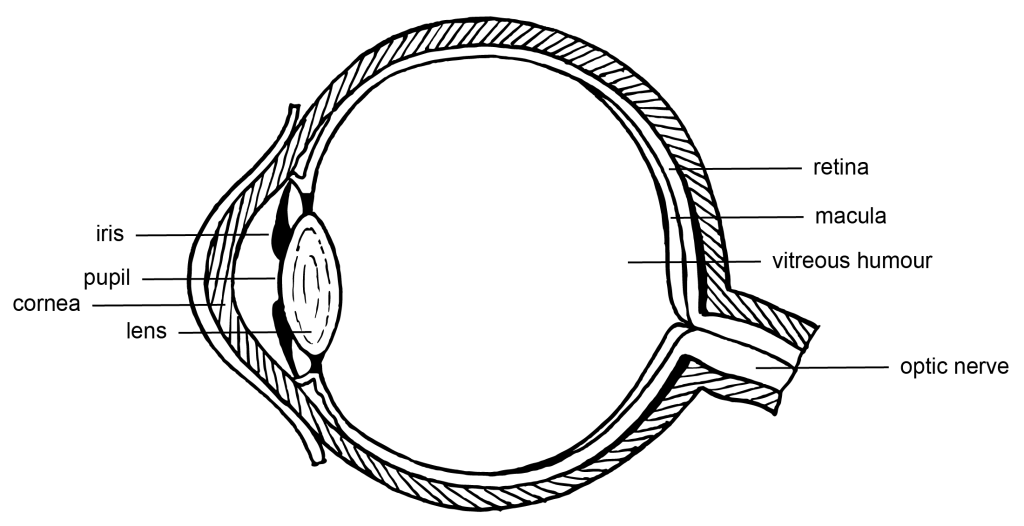
Anatomy of the human eye
The eye works like a Camera Obscura – a small, almost spherical chamber (Figure 2.43) where light enters via the cornea and through a small hole (the pupil). The iris controls how much light enters the eye through the pupil. Light then passes through a lens which can change shape to focus the image. The image is projected through a transparent, gel-like substance (vitreous humour) to the back of the eye (retina and macula), which contains light-sensitive cells. The retina is connected to the brain via the optic nerve.
Just like in a camera obscura, the image projected onto the retina is upside-down and reversed, but we learn to interpret the visual input the right way around.
What is perhaps the most interesting part of eye biology for colour theorists is how the cells in the retina are sensitive to light and colour and how this information is transmitted to the brain.
Retinal cells
Photons of light falling on the light-sensitive cells of the retina are converted into electrical signals that are transmitted to the brain by the optic nerve (Figures 2.44 and 2.45). The brain interprets this information as vision.
There are three types of cells in the retina that convert light energy into electrical energy.
Rods
These cells respond to low-intensity light and help us to see low-resolution, black-and-white images – it’s why we can still see things in very low light. Animals that can see in the dark have more rods than we do.
Cones
These cells respond to high-intensity light and enable us to see high-resolution, coloured images.
Photosensitive ganglion cells
These cells respond to a full range of light intensities and help the eye adjust the amount of light that enters the eye and reaches the retina. Ganglion cells help define what we see – especially when it comes to recognising edges and borders.
Ganglion cells have also been discovered to regulate the hormone melatonin, and the circadian rhythm, which both help us have a regular sleep cycle. This is why it’s not good to look at your screen devices late at night because the blue light stimulates these cells and can inhibit natural sleep processes.
Cones – how do they detect colour?
There are two current theories about how cone cells detect colour, and they can both help us understand how colour vision works.
Trichromatic colour vision theory
Trichromatic theory of colour vision tells us that we have three different types of cone cells in our eyes – one each for red, green and blue. These cells are stimulated by different ranges of light waves, and it’s the combination of information from all three types of cells that makes up the millions of colours our brains can perceive. However, it doesn’t fully explain phenomena like how we can’t see “yellowish-blue” or “reddish-green”.
Opponent and complementary colour theories
The opponent process theory of colour tries to describe how the cone cells are more complicated than just red, green, and blue receptive. Instead, the cones have opposite reactions. Originally the opponent pairs were thought to be red-green, blue-yellow and black-white. But in recent times, complementary colour theory shows us that the three “opponent” pairs are red-cyan, green-magenta, and blue-yellow.
These are very complex theories that attempt to explain all the weird things that we see, like afterimage optical illusions. None of the theories described here are able to explain all of our visual experiences, but research continues to explore how our eyes and brain work together to see colour.
You can test the afterimage and complementary colour theory now – all you need is a white piece of paper or wall and the image in Figure 2.46.
Activity – afterimage optical illusion
Stare at the image in Figure 2.46 for 20 seconds (red square with a smaller white interior square). Then quickly look at a piece of white paper or a white wall and stare at the white area for a while.
What you might briefly see is a faint afterimage of the opposite colours – a blurry cyan square with a smaller dark square inside it, as in Figure 2.47.
This optical illusion can be explained by opponent theory. By staring at the red square for a long time, the cone cells become weaker at detecting red (or transmitting the red vision signal to the brain). Therefore, when you look away to the white paper, the white colour doesn’t register properly as white, it leaves out the red part of white light, and you only see the opposite colour – cyan.
After a while, this illusion fades and your vision will return to normal.
Learn more about opponent process on Wikipedia
Chimerical colours – impossible or imaginary colours.
The afterimage in the experiment above is also known as a chimerical colour, after the mythical beast, the Chimera. Objects around us do not have imaginary colours, we use that term to describe colours we might see that are not part of normal vision. However, theoretically, there are mathematical descriptions of colours that are outside the visual colour space.
The cone cells in our eyes have sensitivities to short, medium and long wavelengths, and these sensitivities overlap a bit. We don’t usually see colour with only one type of cone at a time – it’s usually a mixture. An impossible colour might occur, for example, if we only saw green with the medium-length cone – we might see it as an extremely intense green. Something outside of the range of the CIELAB colour space.
Types of Chimerical colours (Figure 2.48)
Stygian
Stygian colours are both dark and impossibly saturated. You could see “stygian blue” by staring at bright yellow (to cause a dark blue afterimage), then looking at a deep black surface. The blue afterimage is viewed over the black, and simultaneously as dark as the black.
Self-luminous
The experiment using Figure 2.46 is an example of self-luminous colour. The cyan afterimage is self-luminous and might appear even brighter than the white paper you are looking at.
Hyperbolic
This is an impossibly highly saturated colour (hyperbolic means exaggerated). You can try an example of this more intense and highly saturated colour by changing the experiment using Figure 2.46.
Try the experiment again, and this time, after you focus on the red square (Figure 2.46), look straight at the blurry cyan square below it (Figure 2.47). You should see an impossibly bright cyan – brighter than any colour you could see with normal light.
Eye fatigue template for impossible colours
In Greek mythology, a fire-breathing female monster with a lion's head, a goat's body, and a serpent's tail.
Stygian means 'very dark'. The word comes from the River Styx in Greek mythology - which was the way to the underworld.