3.1 The Octet Rule and Lewis Dot Diagrams
Learning Objectives
- Understand the octet rule and how to read and write Lewis dot diagrams
- Utilise Lewis dot diagrams to predict the bonding of simple molecules.
In 2.2 ‘Electronic Configuration’, we discussed how valence electrons are essential to determining the bonding of elements. Generally speaking, elements want to achieve 8 electrons in their outer shell — the same configuration seen in noble gases — and will bond with other elements to make this occur. This rule of thumb is known as the octet rule, and is true for elements up to the [latex]d[/latex] block.
The Lewis electron-dot model is based on the fact that atoms tend to combine to give compounds that achieve a noble gas valence electron configuration of [latex]s^{2}p^{6}[/latex] (or [latex]s^{2}[/latex] for hydrogen). Elements within this model are represented through their chemical symbol and dots, which depict valent [latex]s[/latex] and [latex]p[/latex] electrons. Following the rules of electronic orbitals, electrons are placed surrounding each of the four sides of the element before paring up (see Figure 3.1.1).
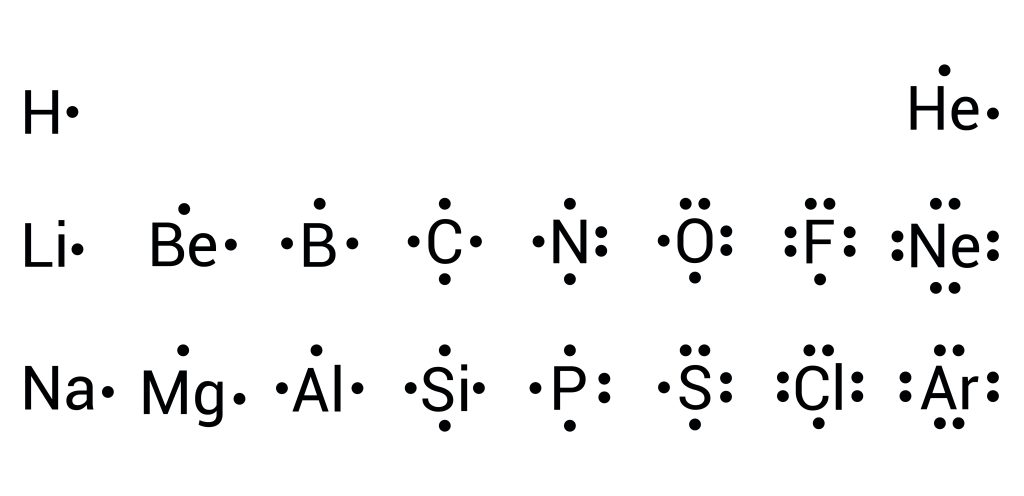
To understand how dot diagrams can help us predict bonding, let’s have a look at a compound containing sodium ([latex]\ce{Na}[/latex]) and chlorine ([latex]\ce{Cl}[/latex]). We will begin by determining the number of valence shell electrons in each:
Atom | Atomic number | Electron configuration | Valence shell electrons |
---|---|---|---|
[latex]\ce{Na}[/latex] | 11 | [latex]1s^{2}2s^{2}2p^{6}3s^{1}[/latex] | 1 |
[latex]\ce{Cl}[/latex] | 17 | [latex]1s^{2}2s^{2}2p^{6}3s^{2}3p^{5}[/latex] | 7 |
Sodium contains 1 valence electron, while chlorine has 7. For both to achieve full valence shells, sodium can donate its electron to chlorine.
The atom that can form most bonds is considered the central atom. If two different atoms can form the same number of bonds, then the least electronegative atom should be the central atom. Among sodium and chlorine, sodium is the least electronegative atom. Let us draw the two elements as Lewis Dot Diagrams (see Figure 3.1.2):
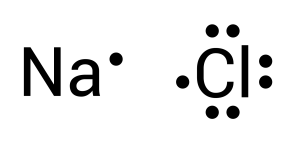
To create this neutral compound, a single bond is formed between sodium and chlorine to donate electrons. This is depicted by combining the two dots together or by drawing a line (see Figure 3.1.3):
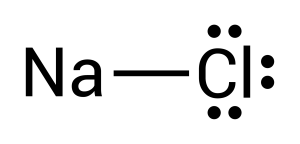
Chemistry Is Everywhere: Salt
The element sodium is a very reactive metal; given the opportunity, it will react with the sweat on your hands and form sodium hydroxide, which is a very corrosive substance. The element chlorine is a pale yellow, corrosive gas that should not be inhaled due to its poisonous nature. Bring these two hazardous substances together, however, and they react to make the ionic compound sodium chloride, known simply as salt. Sodium, chlorine, and sodium chloride can be seen in Figure 3.1.4 below:
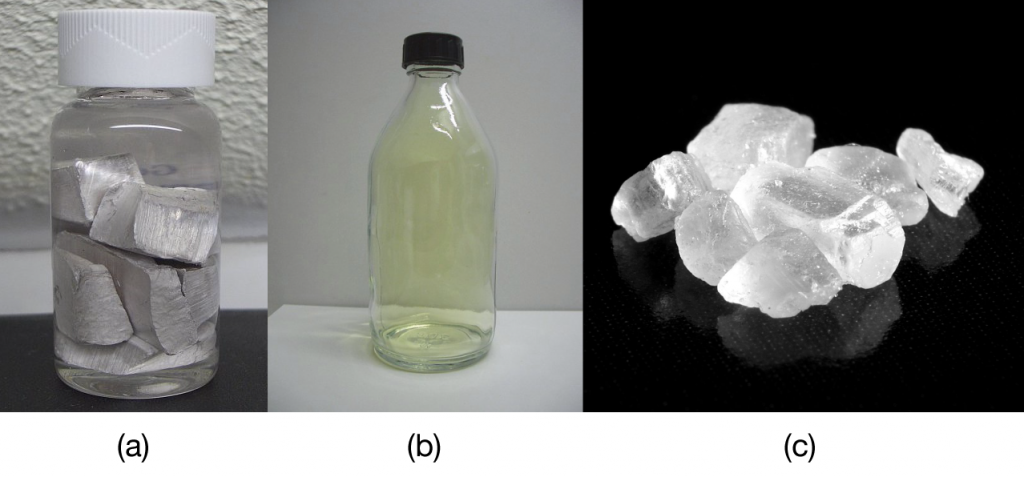
Salt is necessary for life. Na+ ions are one of the main ions in the human body and are necessary to regulate the fluid balance in the body. Cl− ions are necessary for proper nerve function and respiration. Both of these ions are supplied by salt. The taste of salt is one of the fundamental tastes; salt is probably the most ancient flavouring known and one of the few rocks we eat.
The health effects of too much salt are still under debate, although a 2010 report by the US Department of Agriculture concluded that “excessive sodium intake … raises blood pressure, a well-accepted and extraordinarily common risk factor for stroke, coronary heart disease, and kidney disease.”[1] It is clear that most people ingest more salt than their bodies need, and most nutritionists recommend curbing salt intake. Curiously, people who suffer from low salt (called hyponatremia) do so not because they ingest too little salt but because they drink too much water. Endurance athletes and others involved in extended strenuous exercise need to watch their water intake so their body’s salt content is not diluted to dangerous levels.
This completes the diagram for sodium chloride. Let’s have a look at a more complex molecule, ammonia (NH3). Ammonia contains nitrogen and hydrogen. As before, we will begin by considering the valence electrons.
Atom | Atomic number | Electron configuration | Valence shell electrons |
---|---|---|---|
[latex]\ce{H}[/latex] | 1 | [latex]{1s^{1}}[/latex] | 1 |
[latex]\ce{N}[/latex] | 7 | [latex]1s^{2}2s^{2}2p^{3}[/latex] | 5 |
Hydrogen contains 1 valence electron, while nitrogen has 5. To achieve a full valence shell, 3 hydrogens are needed to donate their electrons with nitrogen (see Figure 3.1.5).
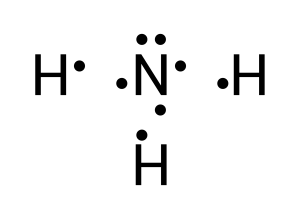
To create this neutral compound, a single bond is formed between each of the hydrogen and the lone nitrogen to donate electrons (see Figure 3.1.6):
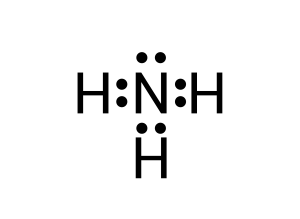
Lewis dot diagrams are vital in understanding the geometry of molecules. As we will explore in VSEPR theory — the location of lone pairs of electrons are of great importance. Let us review one more example: carbon dioxide (CO2).
Atom | Atomic number | Electron configuration | Valence shell electrons |
---|---|---|---|
[latex]\ce{C}[/latex] | 6 | [latex]1s^{2}2s^{2}2p^{2}[/latex] | 4 |
[latex]\ce{O}[/latex] | 8 | [latex]1s^{2}2s^{2}2p^{4}[/latex] | 6 |
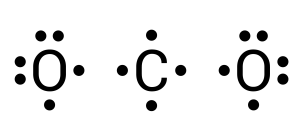
In this compound, carbon is the central atom (see Figure 3.1.7). Carbon requires 4 more electrons to complete its valence shell. We can begin by donating one electron from each neighbouring oxygen atom (see Figure 3.1.8):
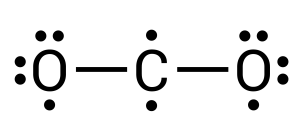
After completing this, we can see how all atoms still have unpaired electrons present. Two on the carbon and one on each of the oxygens. For a stable compound to form, all electrons must be paired. This, therefore, must mean that the oxygens are double bonded to the carbon, where two pairs of electrons are transferred/shared between elements (see Figure 3.1.9). Within chemistry, triple bonds are also a possibility.
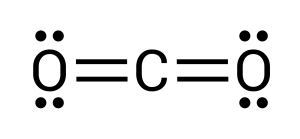
Some molecules do not follow the octet rule. Atoms such as sulphur, phosphorus and chlorine deviate from the octet rule by having more than eight electrons in the valence shell. This is due to the availability of vacant d orbitals in the third shell. These exceptions are fully explored in tertiary chemistry studies.
Key Takeaways
- Main group elements want to achieve 8 electrons in their outer shell. This is known as the octet rule.
- Lewis electron-dot diagrams allow for us to visually represent valent electrons and predict bonding.
- Some molecules require the formation of double and triple bonds to complete their octet.
- Not all compounds follow the traditional octet rule, such as sulphur and phosphorous.
Exercises
Media Attributions
- Lewis dot structure of elements 1 to 18. Periodic table © Zizo - stock.adobe.com is licensed under a All Rights Reserved license
- U.S. Department of Agriculture Committee for Nutrition Policy and Promotion, “Report of the Dietary Guidelines Advisory Committee on the Dietary Guidelines for Americans,” accessed January 5, 2010, https://www.dietaryguidelines.gov/sites/default/files/2019-05/2010DGACReport-camera-ready-Jan11-11.pdf. ↵
Electrons found on the outer shell of an atom.
The trend that atoms like to have eight electrons in their valence shell.
A covalent bond composed of two pairs of bonding electrons.
A covalent bond composed of three pairs of bonding electrons.