3.6 Predicting Molecular Shape
Learning Objectives
- Utilising VSEPR theory, predict the geometries of molecules through the presence of lone-pairs and electron-dense groups.
- Determine the polarity of a molecule, considering bond types and geometry.
You may have noticed that when drawing or representing molecules, we sometimes draw them with unexpected geometry. Some of the most well-known organic molecules, such as methane, formaldehyde and carbon dioxide all have different shapes.
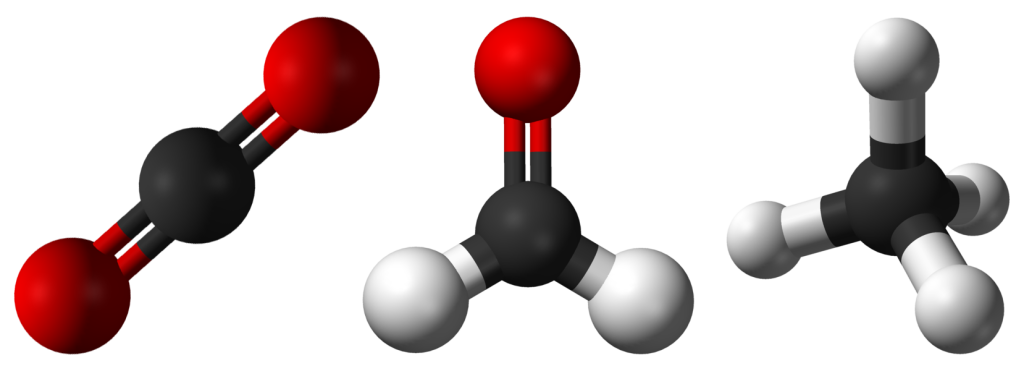
There seems to be sound reasoning behind this as all three compounds have different numbers of atoms present. However, consider carbon dioxide compared to a molecule of water; even though both molecules have the same number of atoms, we represent them differently. Water is found to have a bent structure, while carbon dioxide is perfectly linear (see Figure 3.6.2); why is this the case?
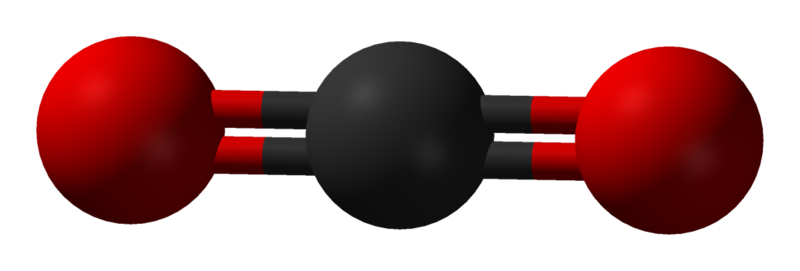
VSEPR Theory
To understand why certain molecules present in this way, let us consider the repulsive effect of electrons through the Valence Shell Electron-pair Repulsion model, also known as VSEPR theory. Recall that electrons have negative charges. Electrons will, therefore, emit repelling forces upon each other and will try to roughly position themselves where the minimum repulsive force is experienced. By understanding how many high-density regions of electrons exist, we can best spread them out and predict geometry.
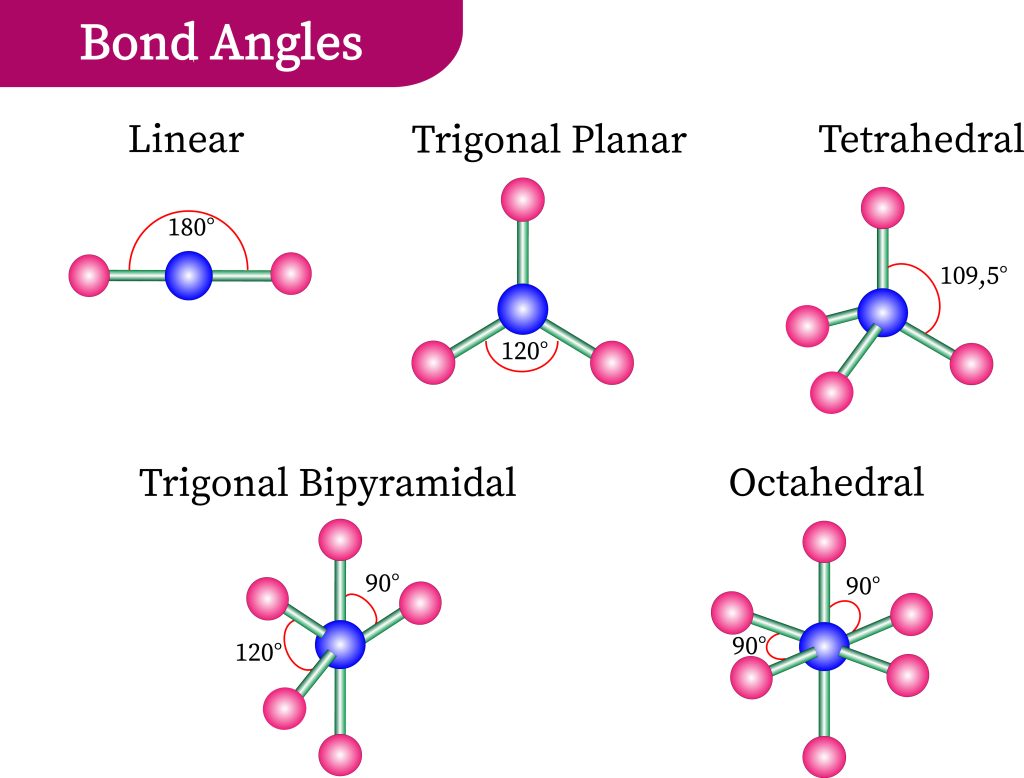
In Figure 3.6.3, we observe the preferred orientation of molecules depending on the number of electron-dense regions. The proposed shapes have all electron-dense regions positioned as far as possible from each other. These regions can either be bond pairs or lone electron pairs (on the central atom). In the case of carbon dioxide, we have two regions of high-electron density, each found at the bonds between the central carbon and oxygen atoms present. As such, a linear formation naturally occurs (see Figure 3.6.4).
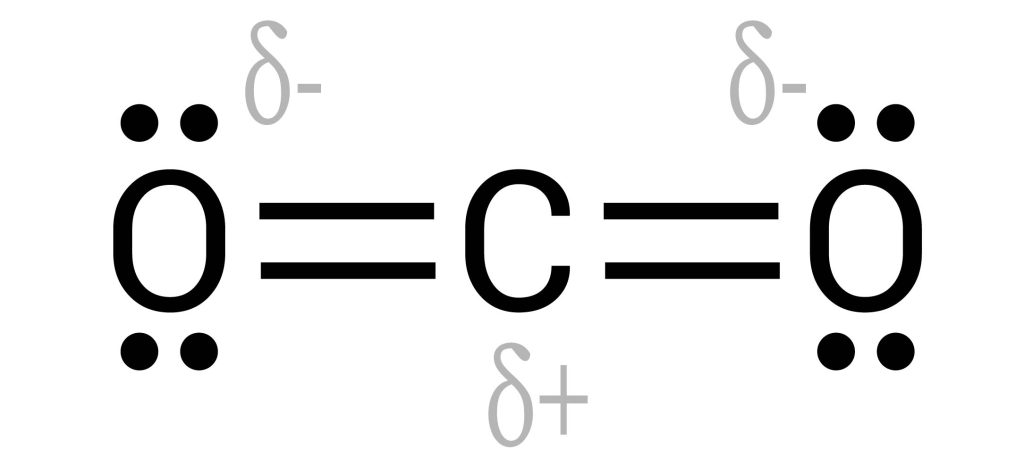
In the case of water, however, we detect the two electron-dense groups from the two O-H bonds and the two lone pairs of electrons on the oxygen atoms. This means there are four regions of electron density — inferring that the preferred configuration would be a tetrahedral arrangement. Naturally, however, the paired electrons do not generate bonds of their own. Removing two of the electron-dense regions produces a final ‘bent’ or angular geometry (see Figure 3.6.5).
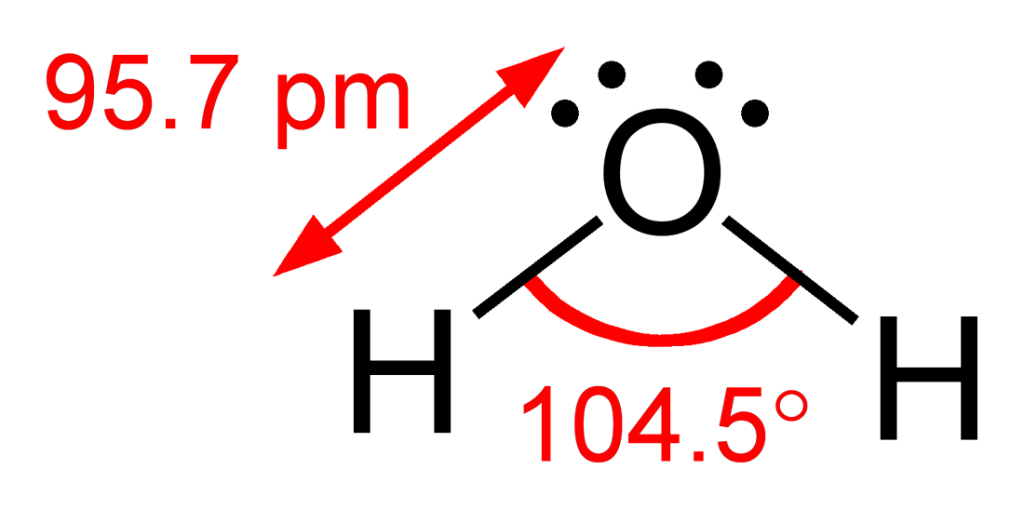
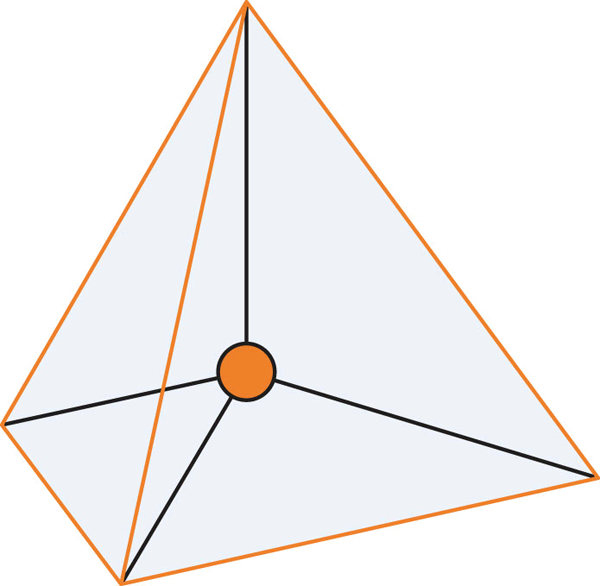
As such, it is important to note that in VSEPR theory, all molecules take up their preferred orientations – however, the presence of lone pairs makes this appear as if it does not happen. For instance, methane, ammonia and water all have 4 regions of electron density and form tetrahedral arrangements (see Figure 3.6.6). Methane is able to accomplish this, while nitrogen (with one lone pair of electrons) forms a trigonal pyramid without another hydrogen bond. Water (with two lone pairs of electrons) forms a “bent” geometry – there is a clear angle between the two bonds.
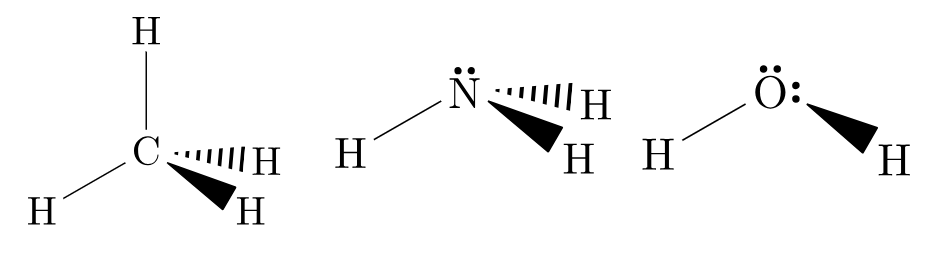
Therefore, the overall geometry of the molecule can be categorised by the number of atoms and the number of lone electron pairs present on the central atom. Figure 3.6.8 details a helpful guide to predict shape and can be relied upon as a general rule for most molecules. Let’s use the table to help us ascertain the geometry of another organic molecule: formaldehyde.
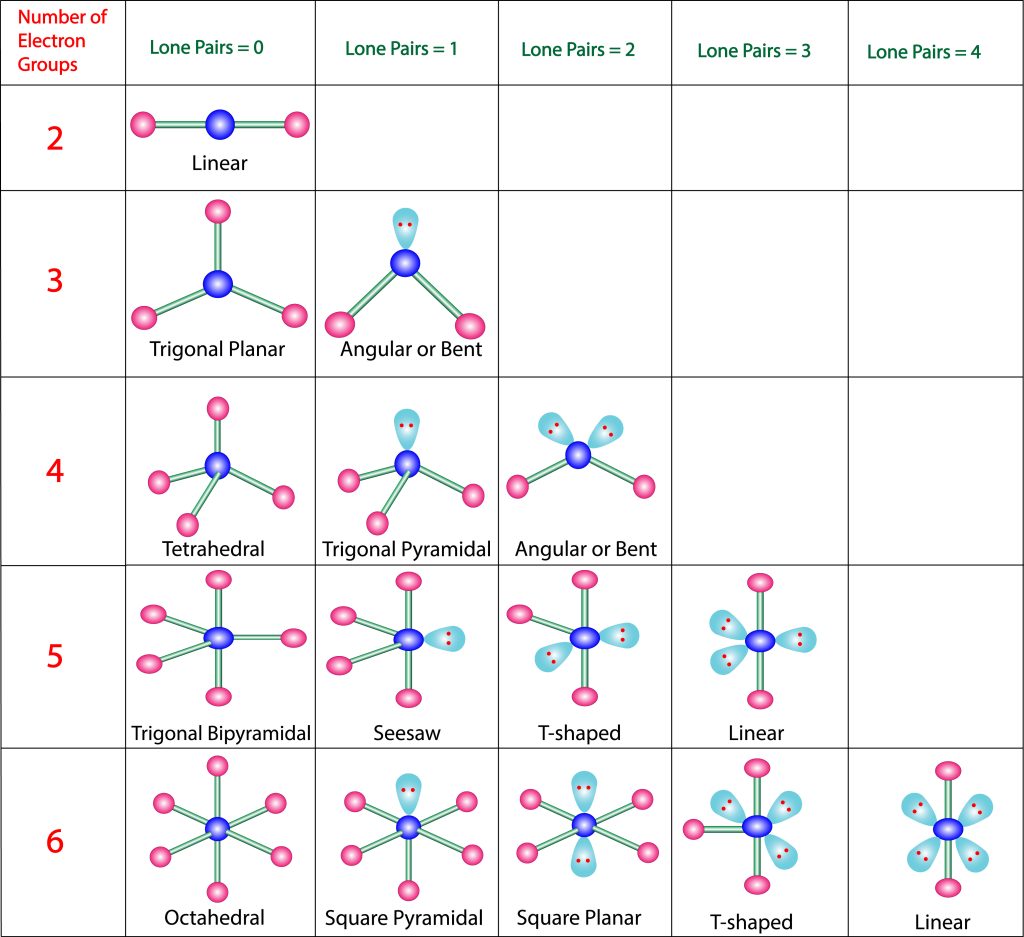
Example
VSPER theory represents an idealised set of interactions between electron-dense areas within a molecule. Bond angles and lengths differ slightly between molecules dependant on the types of elements within. However, they are a great generalisation and should be used moving forward in undergraduate studies. Have a look at the simulation below for how real molecules differ to the model predictions.
Simulation by PhET Interactive Simulations, University of Colorado Boulder, licensed under CC-BY-4.0 (https://phet.colorado.edu).
Now that we understand the geometry of molecules, we can begin to determine if a molecule is polar or non-polar. Recall that these terms were used when discussing bond formation. Polarity refers to the presence of a (whole or partial) magnetic charge generated through atomic interactions, which can be determined through a difference in electronegativity.
Carbon dioxide is a linear molecule consisting of two double-bonded C=O’s. These bonds are polar covalent due to their difference in electronegativity. The carbon is electropositive, while the oxygens are electronegative. You would therefore expect the entire molecule to be considered polar. However, if we draw out these negative forces as charges, they directly oppose each other – cancelling each other out, as seen in Figure 3.6.9.
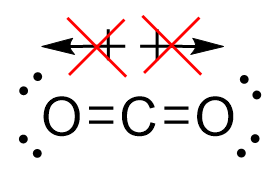
As such, while there are polar covalent bonds with electronegative ends, the entire molecule does not experience a net charge. This makes carbon dioxide non-polar.
Conversely, for water, the O-H bonds are also polar covalent. However, the introduction of the angular bend means that the forces experienced are not directly opposing each other. While the horizontal components of the force do cancel each other out, the vertical vectors ensure that a permanent dipole is present, meaning that the molecule is polar.
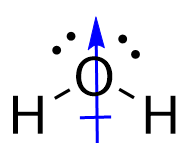
To understand how the polarity of a molecule is determined by the electronegativity of atoms, have a look at the following interactive activity’s “Three Atoms” simulation with partial charges enabled:
Simulation by PhET Interactive Simulations, University of Colorado Boulder, licensed under CC-BY-4.0 (https://phet.colorado.edu).
Key Takeaways
- VSEPR Theory allows for the prediction of molecule geometry according to the number of electron-dense regions and lone pair electrons present.
- Molecular geometry can result in certain molecules, which feature polar bonds, to be non-polar due to cancelling out forces.
Exercises
Media Attributions
- Vector illustration of Bond Angles © aboabdelah - stock.adobe.com is licensed under a All Rights Reserved license
- vector illustration of VSEPR Theory CHART. © aboabdelah - stock.adobe.com is licensed under a All Rights Reserved license
Valance shell electron-pair repulsion model; used to predict molecular geometry through regions of high electron density.
A molecule or bond that experiences a (partial) charge.
A molecule or bond that does not experiences a (partial) charge.