3.3 Covalent Bonds and Polarity
Learning Objectives
- Describe a non-polar bond and a polar bond.
- Use electronegativity to determine whether a bond between two elements will be nonpolar covalent, polar covalent, or ionic.
While ionic bonds form when metals donate electrons to non-metals, covalent bonds are formed between two non-metals sharing electrons. As a result of this, covalent bonds form when the difference in electronegativity between elements is below 2. Covalent bonds are the strongest kind of bond that can be formed. Atoms held together by covalent interactions give rise to molecular compounds.
Similar to ionic compounds, atoms involved in a covalent bond acquire the octet in their valence shell by sharing electrons between atoms. Generally, the number of covalent bonds an atom forms depends on how many electrons an atom requires to achieve an octet. For instance, [latex]\ce{C}[/latex] forms four covalent bonds as the electronic configuration ([latex]1s^{2}2s^{2}2p^{2}[/latex]) needs four more electrons to acquire an octet in the valence shell.
Carbon’s ability to form 4 covalent bonds makes it a vital element — with some regard it as the most important element in life. Carbon’s prevalence within many molecules is detailed in the subject of organic chemistry — which explores the synthesis of a variety of compounds and their functional groups.
Polarity
Covalent bonds are formed through the sharing of electrons. The difference in electronegativity is still, however, important, as it indicates if the shared electron can be found closer to one atom or another. Let’s observe how this presents in hydrogen fluoride (see Figure 3.3.1):
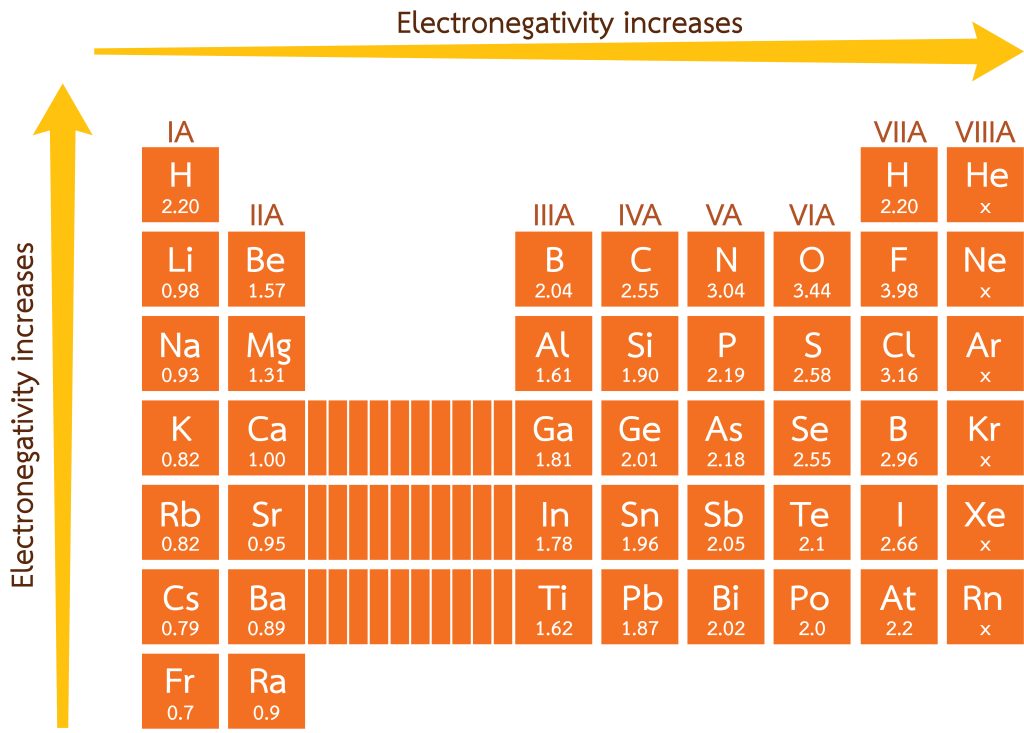
Utilising the electronegativity values presented in Figure 3.3.2, [latex]\ce{H}[/latex] is valued at 2.20, where [latex]\ce{F}[/latex] presents at 3.98. The difference in electronegativity is 1.78. While this isn’t enough to qualify as an ionic bond, the higher electronegativity on the fluoride atom causes the electron to be positioned in space closer towards it. This generates a partial charge, represented by a lowercase delta (δ). The more electronegative atom gains a partial negative charge due to the electron being closer to the atom, while the less electronegative generates a partial positive (see Figure 3.3.3). The presence of these partial charges gives rise to a very important chemical concept: polarity. These types of bonds, such as the one in hydrogen fluoride, are known as polar covalent bonds.
The net polarity of a molecule depends on the polarity of the individual bonds, the contribution from lone pairs, and the molecules’ shape. In a polar molecule, electrons are more strongly attracted to one part of the molecule than the other. For example, in the water molecule, due to the high electronegativity of the oxygen atom, electrons are more strongly attracted towards the oxygen atom than they are to the hydrogen atom. In some cases, although the individual covalent bonds are polar, the overall molecule becomes non-polar due to the shape of the molecule. For instance, the carbon dioxide molecule has polar covalent [latex]\ce{C}-\ce{O}[/latex] bonds. However, due to the linear symmetrical shape of the molecule, polar bonds (dipoles) cancel each other out, creating a zero net polarity (see Figure 3.3.4).
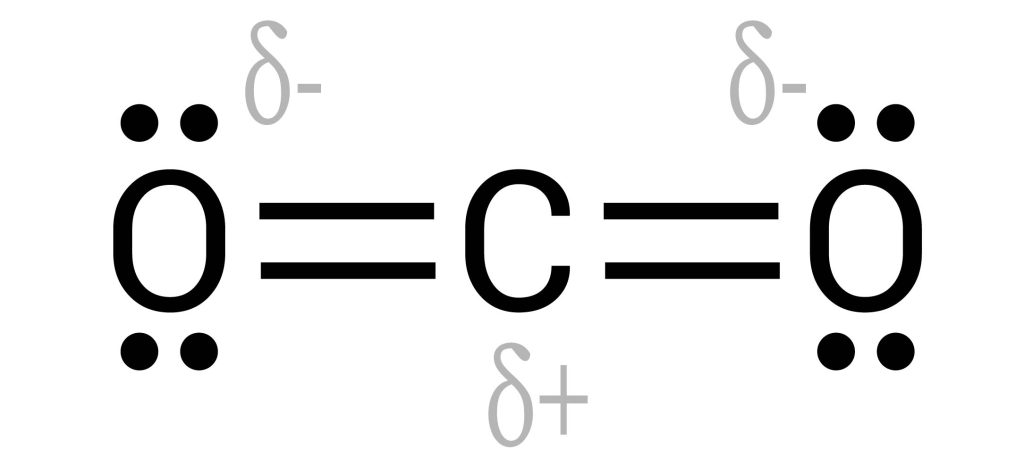
A lot of elements within nature that are gases at room temperature also form covalent bonds with themselves in order to complete their octets. [latex]\ce{H_2}[/latex], [latex]\ce{N_2}[/latex] and [latex]\ce{O_2}[/latex] are some examples (see Figure 3.3.5). With the difference in electronegativity between the two atoms being 0 (as they are the same), the type of bond that forms is a non-polar covalent bond.
The net polarity of a molecule has implications regarding melting/boiling points, viscosity and, most importantly, solubility. We will discuss how to determine a molecule’s polarity more during 3.6 Predicting Molecular Shape. For now, we can identify polar covalent from normal covalent bonds through the difference in electronegativity detailed in Table 3.3.1.
Electronegativity difference | Type of bond | Examples |
---|---|---|
>2.0 | Ionic | [latex]\ce{NaCl}[/latex], [latex]\ce{CsF}[/latex] |
0.5-1.9 | Polar covalent | [latex]\ce{HCl}[/latex], [latex]\ce{HF}[/latex] |
0-0.4 | (non-polar) Covalent | [latex]\ce{CH}_{4}[/latex], [latex]\ce{O}_{2}[/latex], [latex]\ce{H}_{2}[/latex] |
To understand how the polarity of a bond differs with electronegativity, have a look at the following interactive activity’s “Three Atoms” simulation with partial charges enabled:
Simulation by PhET Interactive Simulations, University of Colorado Boulder, licensed under CC-BY-4.0 (https://phet.colorado.edu).
Naming Binary Molecular Compounds
To name covalent compounds with 2 elements, take the name of the less electronegative atom first, followed by the more electronegative atom. Therefore, metals come before nonmetals and nonmetals located towards the left side of the periodic table. Additionally, the name of the more electronegative atom should end with the suffix -ide as used for anions. Depending on the number of atoms of each element involved in the compound, prefixes such as di-, tri-, tetra- and penta- are utilised (see Table 3.3.2). For instance, the compound [latex]\ce{CCl}_{4}[/latex] is named as “carbon tetrachloride”.
Molecular formula | Name of the compound |
---|---|
[latex]\ce{CO}[/latex] | Carbon monoxide |
[latex]\ce{CO}_{2}[/latex] | Carbon dioxide |
[latex]\ce{BBr}_{3}[/latex] | Boron tribromide |
[latex]\ce{N}_{2}\ce{O}_{5}[/latex] | Dinitrogen pentoxide |
Key Takeaways
- Covalent bonds form between two non-metals sharing electrons.
- Covalent bonds are identified as having a difference in electronegativity lower than 2.
- When the difference in electronegativity is between 0.4-2, a polar covalent bond forms. This bond has partial charges, with the δ– assigned to the more electronegative element.
- Binary compounds are named by the less electronegative element first, followed by the other element involved. Prefixes are added to denote how many of each element are present.
Exercises
Media Attributions
- Periodic table of elements © Torsu - stock.adobe.com is licensed under a All Rights Reserved license
The attraction between oppositely charged ions.
A chemical bond formed by two atoms sharing electrons.
Power of an atom to attract electrons; determines bond formation.
A slight positive or negative charge on a polar covalent compound.
The presence of (magnetic) poles.