8.7 Types of Organic Reactions
Learning Objectives
- Describe different reaction types in organic chemistry.
- Predict the products obtained upon reactions carried out by hydrocarbons, alkyl halides and alcohols.
The practice of organic chemistry includes understanding, planning for and carrying out functional group transformations of these types. A knowledgeable organic chemist can link together reactions to build specific target molecules according to a plan. Organic chemical synthesis has applications in many industries, from food to pharmaceuticals, adhesives and coatings, and more.
Main Reaction Types in Organic Chemistry
Organic chemistry involves a wide variety of reactions, each with its own set of conditions and mechanisms. Reaction types can be categorised based on the changes that occur in the structure of the molecules involved.
- Addition Reaction: In addition reactions, atoms or groups of atoms are added, typically to a double or triple bond. In these reactions, one of the bonds in the double bond is broken. Each of the carbon atoms in the bond can then attach another atom or group while remaining joined to each other by a single bond. An example is the reaction between ethene and bromine. (See Figure 8.7.1). In this reaction, bromine atoms are added across the carbon-carbon double bond in ethene. The addition reaction with bromine can be used to test for alkenes in an uncharacterised sample of material. Bromine solutions are brownish-red. When we add a [latex]\ce{Br_{2}}[/latex] solution to an alkene, the colour of the solution disappears because the alkene reacts with the molecular bromine.
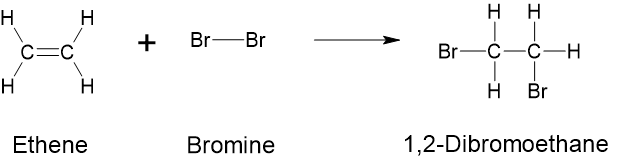
- Substitution Reactions: Substitution reactions involve the replacement of one functional group or atom by another. Let’s consider the reaction between bromomethane ([latex]\ce{CH_{3}Br}[/latex]) and hydroxide ion ([latex]\ce{OH^{-}}[/latex]) as shown in the following equation. The bromine atom in bromomethane is replaced by the hydroxide ion, forming methanol and leaving the bromide ion.
[latex]\ce{CH_{3}Br} + \ce{OH^{-}} {\rightarrow} \ce{CH_{3}OH} + \ce{Br^{-}}[/latex]
- Elimination Reactions: Elimination reactions involve the removal of elements from a molecule, leading to the formation of a double bond or a triple bond. This is the opposite of addition reactions. An example of an elimination reaction is shown below in Figure 8.7.2. Here, the bromine atom attached to the fourth carbon and one of the hydrogens attached to the adjacent carbon (third carbon in this case) leave, forming a double bond between the third and fourth carbons.
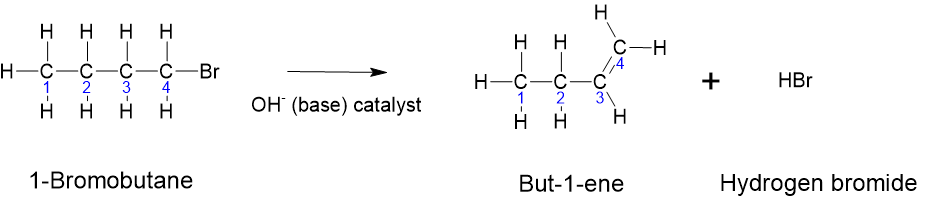
- Oxidation and Reduction Reactions: As you learned in Chapter 4.6 (red-ox reactions), reduction is the gain of electrons, and oxidation is the loss of electrons of an element or atom. The same principle applies in organic chemistry as well. Nevertheless, due to the intricate nature of organic molecules, organic chemists use the following conventions to identify oxidation and reduction reactions:
- Oxidation is when there is an increase in the number of [latex]\ce{C}-\ce{O}[/latex] bonds and or a decrease in the number of [latex]\ce{C}-\ce{H}[/latex] bonds. Figure 8.7.3 displays the oxidation of alcohol into aldehyde and further oxidation leading to the production of carboxylic acid. You can see that the number of [latex]\ce{C}-{O}[/latex] bonds increase when ethanol converts to acetaldehyde and acetaldehyde converts to acetic acid.
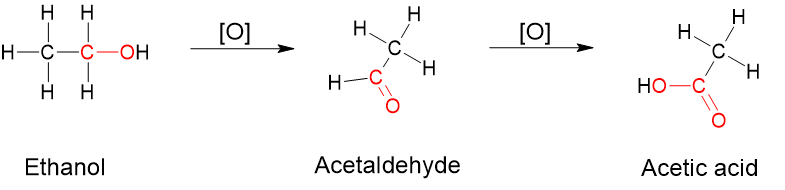
-
- Reduction is the opposite of oxidation. A decrease in the number of [latex]\ce{C}-\ce{O}[/latex] bonds and or an increase in the number of [latex]\ce{C}-\ce{H}[/latex] bonds is considered a reduction. We often observe reduction reactions as the addition of hydrogen across a double or triple bond, as shown in Figure 8.7.4. Reduction reactions are a specific instance of an addition reaction.
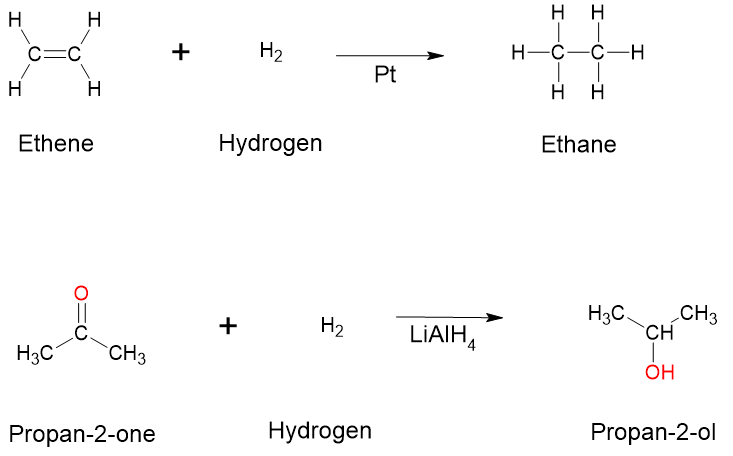
- Condensation Reactions: Condensation reactions refer to a class of reactions in which two molecules combine to form a larger molecule, often with the elimination of a smaller molecule, such as water or alcohol.
- One of the well-known condensation reactions is the esterification reaction between a carboxylic acid and an alcohol. In this reaction, a water molecule is eliminated, and an ester is formed. For example, the esterification of acetic acid with ethanol results in the formation of ethyl acetate and water, as illustrated in Figure 8.7.5.
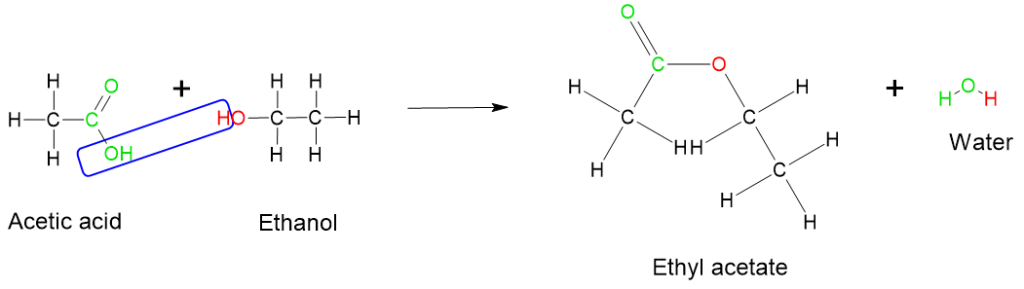
-
- Condensation reactions also occur when amines react with carboxylic acids or acid chlorides, producing an amide ( see Figure 8.7.6). In the example below, acetic acid reacts with ammonia to produce acetamide, leaving a water molecule. In a protein, individual amino acids are joined together by amide bonds. The amide bond, also known as a peptide bond, is formed through a condensation reaction between the carboxyl group of one amino acid and the amino group of another amino acid. This reaction involves the elimination of a water molecule.
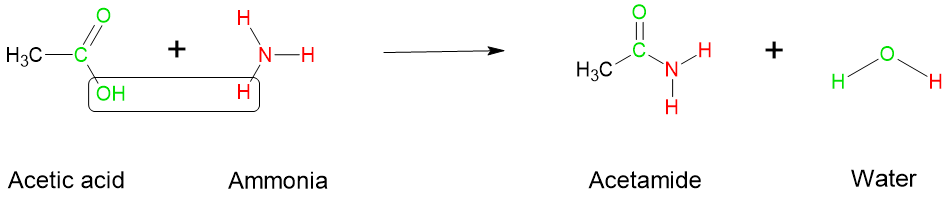
- Hydrolysis Reactions: Hydrolysis reactions involve the cleavage of a bond with the addition of water. This process often results in the breakdown of larger molecules into smaller components. Esters and amides can be reveres into their original reactants by a hydrolysis reaction, as shown in Figure 8.7.8. Hydrolysis is the opposite of the condensation reactions.
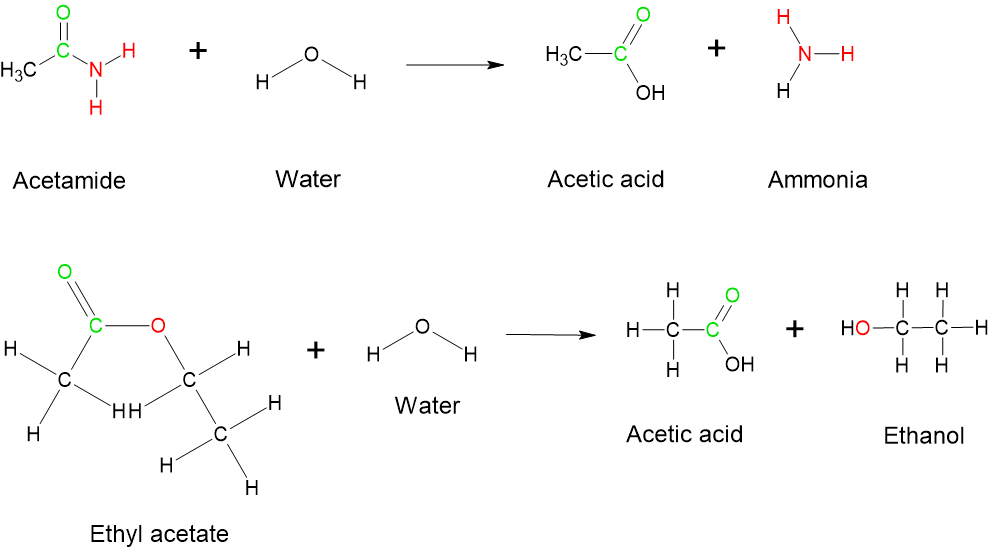
Reactions of Hydrocarbons
Reactions of alkanes
As we learned in Chapter 8.1, alkanes are relatively unreactive compared to other classes of organic compounds due to the non-polar nature and strength of carbon-carbon and carbon-hydrogen single bonds. The only major reactions that alkanes undergo are combustion and substitution reactions.
- Combustion reactions: in combustion reactions, alkanes react with oxygen to produce carbon dioxide and water, as shown in the following chemical equation for methane. This reaction releases a significant amount of energy and is the basis for the use of hydrocarbons as fuels. Methane is the main natural gas used in home heating and cooking.
[latex]\ce{CH_{4}} + \ce{O_{2}} {\rightarrow} \ce{CO_{2}} + \ce{H_{2}O}[/latex]
- Substitution reactions: substitution reactions of alkanes involve the replacement of one or more hydrogen atoms in an alkane with other atoms or groups. The most common type of substitution reaction for alkanes is halogenation, where hydrogen atoms are replaced by halogen atoms (chlorine, bromine, etc.). This process typically occurs under conditions of high temperature or ultraviolet (UV) light. Chloromethane, which is a general anaesthetic, can be produced by the reaction between chlorine and ethane under high temperatures, as displayed in the following equation.
[latex]\ce{CH_{3}CH_{3}}{(g)} + \ce{Cl_{2}}{(g)} {\rightarrow} \ce{CH_{3}CH_{2}Cl}{(g)} + \ce{HCl}{(g)}[/latex]
Reactions of alkenes and alkynes
As alkenes and alkynes are unsaturated hydrocarbons, they are more reactive than alkanes.
Alkenes and alkynes also undergo combustion reactions to form carbon dioxide and water as alkanes:
[latex]\ce{C_{2}H_{4}} + \ce{O_{2}} {\rightarrow} \ce{2CO_{2}} + \ce{2H_{2}O} [/latex]
[latex]\ce{C_{2}H_{2}} + \ce{5H_{2}O} {\rightarrow} \ce{4CO_{2}} + \ce{2H_{2}O}[/latex]
Except for combustion reactions, nearly all other reactions of alkenes and alkynes often involve the breaking of double or triple bonds to form new bonds by addition reactions. The different types of addition reactions of alkenes and alkynes are discussed below.
- Hydrogenation (addition of hydrogen): The simplest addition reaction is hydrogenation. This is a reaction in which hydrogen gas reacts at a carbon-to-carbon double or triple bond to add hydrogen atoms to carbon atoms. In the laboratory, this reaction can be facilitated with hydrogen in the presence of a catalyst such as nickel ([latex]\ce{Ni}[/latex]) or platinum ([latex]\ce{Pt}[/latex]). Examples of hydrogenation reactions are displayed in Figure 8.7.9. The hydrogenation reaction is a specific type of addition reaction, which is also a reduction reaction, as discussed in reduction reactions earlier in this section. Hydrogenation is used to convert unsaturated vegetable oils to saturated fats. Hydrogenated fats, or partially-hydrogenated fats, often appear on nutrition labels for processed foods such as crackers or chips.
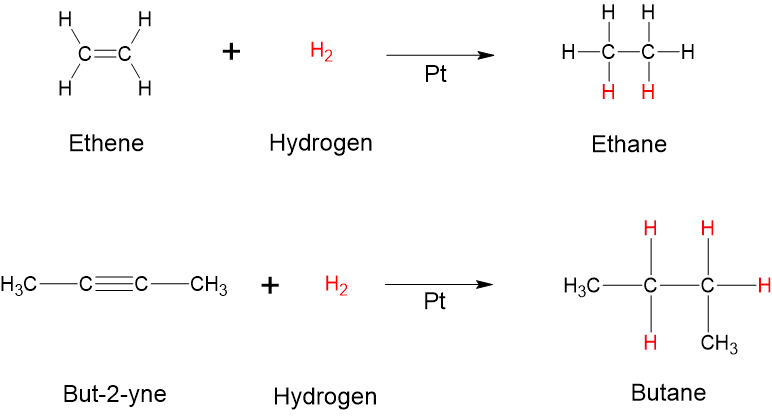
- Halogenation (addition of halogens such as chlorine and bromine): alkenes and alkynes also readily undergo halogenation through addition, a reaction in which a halogen (chlorine or bromine) reacts at a carbon-to-carbon double or triple bond to add halogen atoms to carbon atoms. The reaction involves the addition of one halogen atom to each carbon atom of the double bond or two halogen atoms to each carbon atom of a triple bond (Figure 8.7.10).
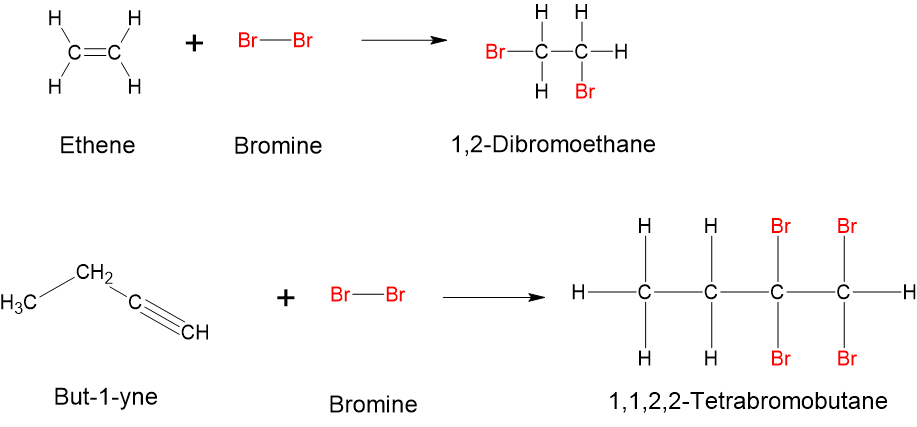
- Hydrohalogenation (Addition of hydrogen halides HX): Alkenes and alkynes react with hydrogen halides such as [latex]\ce{HCl}[/latex], and [latex]\ce{HBr}[/latex] to produce alkyl halides ([latex]\ce{RX}[/latex]). The hydrogen and the halogen atoms are added across the double or triple bond. The following equation displays the addition of hydrogen chloride to ethene.
[latex]\ce{H_{2}C=CH_{2}} + \ce{HCl} {\rightarrow} \ce{CH_{3}CH_{2}Cl}[/latex]
- Hydration (addition of water): Another important addition reaction occurs between an alkene and water to form an alcohol. This reaction is called hydration and represents the addition of water to a substance. In this reaction, water is added across the double bond in the presence of an acid catalyst. The following figure displays an example of a hydration reaction of an alkene.
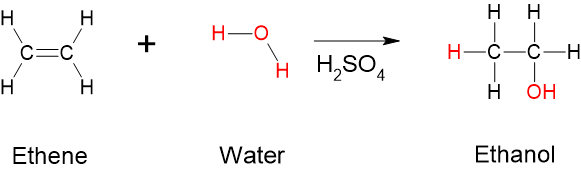
Example 8.7.1
Problem
What are the possible products of the addition of the following reagents to propene [latex]\ce{CH_{2}=CH_CH_{3}}[/latex]:
- [latex]\ce{HCl}[/latex]
- [latex]\ce{H_{2}O}[/latex]
Solution
- The addition of [latex]\ce{HCl}[/latex] is a hydrohalogenation. One carbon atom of the double bond receives hydrogen, and the other carbon in the double bond receives the chlorine atom. Based on this definition, two products are possible, as shown in the following figure. In the first product, the chlorine atom is bound to the first carbon, and in the second product, the chlorine atom is bound to the second carbon.
So, how do we decide which product is actually formed? When unsymmetrical alkenes undergo hydrohalogeneation and hydration reactions, more than one product is possible. Unsymmetrical alkenes means an alkane in which the two carbon atoms of the double bond are not equivalently substituted. For instance, in propene, the first carbon of the double bond has two hydrogens, and the second carbon of the double bond has one hydrogen and one methyl group.
Therefore, in the above reaction, both products are possible to form in reality. In such situations, one product is generally dominant over the other. The dominant product is called the ‘major product’ of the reaction. The major product can be predicted by Markovnikov’s rule. You will learn how to use Markovnikov’s rule to predict products of unsymmetrical alkenes in your university organic chemistry course. As this book is an introductory chemistry book, Markovnikov’s rule will not be covered in this book. If you are interested to know more about Markovnikov’s rule, visit this webpage.
2. The same concept as above applies to the hydration of alkenes. The possible products are [latex]\ce{CH(OH)=CH-CH_{3}}[/latex] and [latex]\ce{CH_{2}=C(OH)-CH_{3}}[/latex], as shown in the following figure.
Addition polymerisation of alkenes
Addition polymerisation is a process in which monomers with unsaturated double bonds react to form a polymer without the elimination of any byproducts. The reaction involves the successive addition of monomers to the growing polymer chain. Addition polymerisation is characteristic of alkenes and results in the formation of polymers with saturated carbon backbones. Common examples of addition polymerisation include the polymerisation of ethene (ethylene) to form polyethylene (Figure 8.7.12) and the polymerisation of propene (propylene) to form polypropylene. The resulting polymers have high molecular weights and find extensive use in various applications due to their physical and chemical properties.
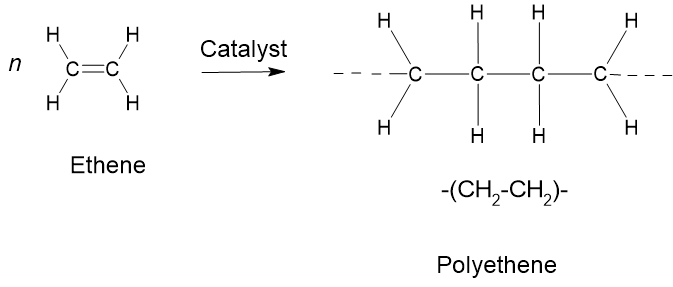
Reactions of functional groups
Reactions of alkyl halides
Alkyl halides, also known as haloalkanes, are compounds in which one or more hydrogen atoms in an alkane have been replaced by halogen atoms (fluorine, chlorine, bromine, or iodine). Alkyl halides differ in polarity compared to alkanes due to the presence of a halogen atom. In alkyl halides, a halogen atom is attached to a carbon atom in place of a hydrogen atom. Halogens are more electronegative than carbon, leading to a significant electronegativity difference. This electronegativity difference results in a polar covalent bond between carbon and the halogen. Therefore, the halogen end of the molecule carries a partial negative charge ( ), and the carbon end carries a partial positive charge ( ). Hence, the carbon atom with a partial positive charge is attacked by the negative ions. As a result, alkyl halides undergo substitution reactions, as shown below in Figure 8.7.13. For instance, the chlorine atom in chloromethane is replaced by a hydroxyl group, forming methanol. Alcohols can be prepared by using reactions between alkyl halides and a base. Alkyl halides undergo substitution reactions with many groups, such as ammonia, amines, cyanide, hydrosulphide and water.
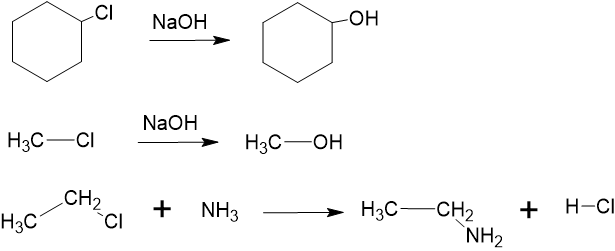
Reactions of alcohols
Alcohols mainly undergo substitution and oxidation reactions.
- Substitution reactions: alcohols can be converted to alkyl halides (alkyl bromides, alkyl chlorides, etc.) through substitution reactions. Primary and secondary alcohols are converted to alkyl halides using thionyl chloride [latex]\ce{SOCl_{2}}[/latex], phosphorus tribromide [latex]\ce{PBr_{3}}[/latex] or phosphorus trichloride [latex]\ce{PCl_{3}}[/latex]. Tertiary alcohols are converted into alkyl halides using [latex]\ce{HCl}[/latex] or [latex]\ce{HBr}[/latex].
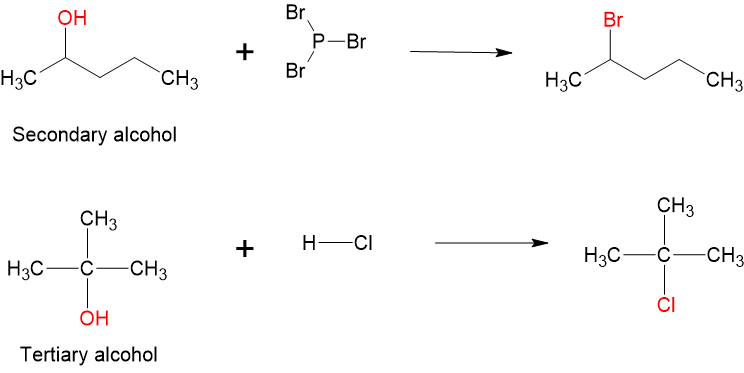
- Oxidation reactions (Figure 8.7.15):
- Primary alcohols can be oxidised to form aldehydes and then further oxidised to carboxylic acids. Mild oxidising agents such as pyridinium chlorchromate are used to convert a primary alcohol to an aldehyde. Common oxidising agents such as potassium permanganate [latex]\ce{KMnO_{4}}[/latex] and potassium dichromate [latex]\ce{K_{2}Cr_{2}O_{7}}[/latex] convert primary alcohol to carboxylic acid.
- Secondary alcohols can be oxidised to form ketones. Further oxidation is not generally favourable.
- Tertiary alcohols are resistant to oxidation under normal conditions.
- Dehydration (Figure 8.7.15): alcohols can undergo dehydration to form alkenes in the presence of heat and an acid catalyst.
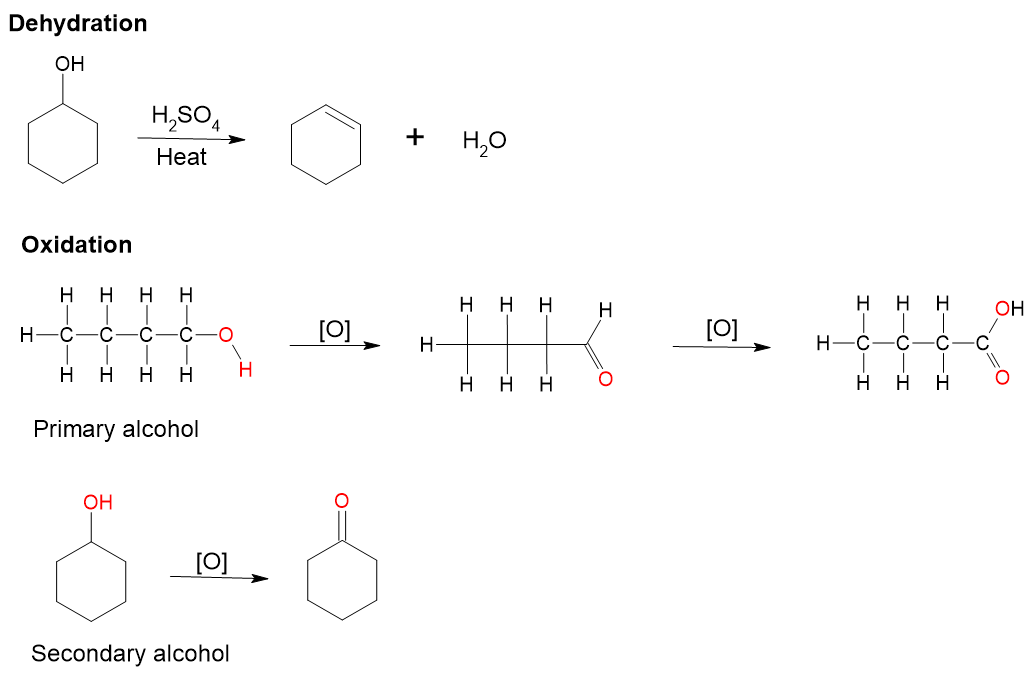
Key Takeaways
- Organic chemistry involves comprehending, planning, and executing functional group transformations, allowing skilled chemists to link reactions and construct targeted molecules systematically.
- Major reaction types in organic chemistry include addition, elimination, substitution, oxidation, reduction, condensation and hydrolysis.
- Due to the less reactivity of alkanes, they only undergo combustion and substitution reactions.
- Alkenes and alkynes undergo different types of addition reactions, including hydrogenation, halogenation and hydrohalogenation.
- The polarity of alkyl halides makes them undergo substitution reactions.
- Alcohols primarily undergo substitution and oxidation reactions.
Exercises
Practice Questions
Predict the products of the following oxidation reactions of alcohols with oxidising agents.
The halogens are elements present in Group VIIA of the periodic table (the group immediately before the Noble gases. Examples of halogens are fluorine, chlorine, bromine and iodine.
an alkane in which the two carbon atoms of the double bond are not equivalently substituted
Power of an atom to attract electrons; determines bond formation.