5.5 Standard Lab Conditions and the Ideal Gas Law
Learning Objectives
- Gain an appreciation of the need for replicability within scientific investigations.
- Define standard laboratory conditions (SLC) and the expected variance between experimental locations.
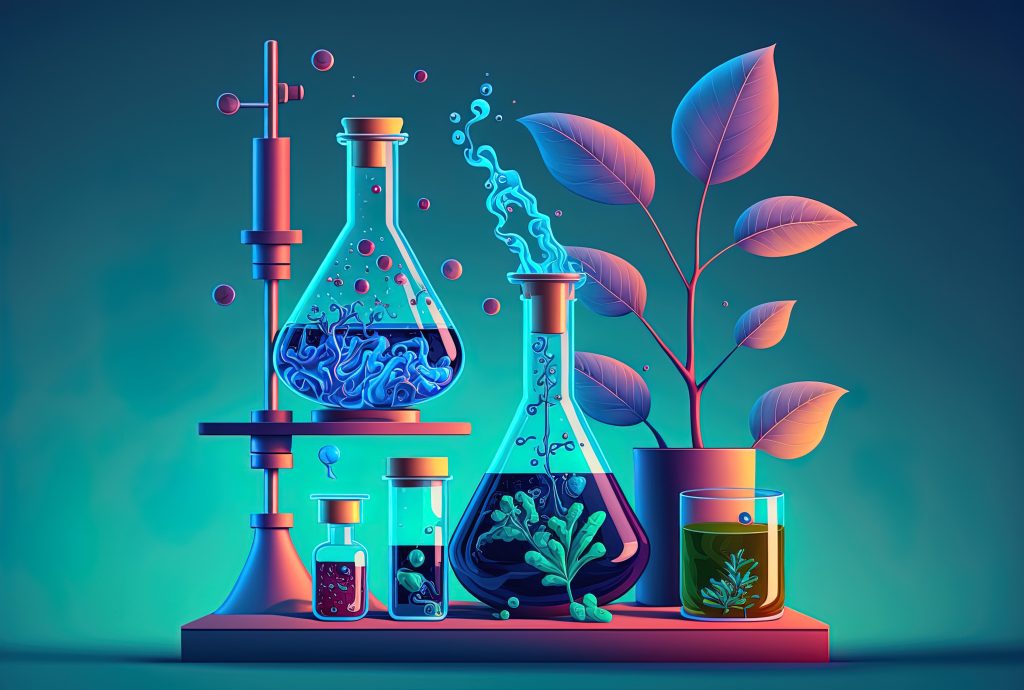
The exact way that reactions process is highly dependent on the environmental conditions in which they occur. While the last chapter, 5.4 ‘Limiting Reagents and Yield’, concerned the amount of reactants present, this understanding can also be applied to the forces and energy experienced by molecules. Temperature and pressure are two common factors that must be considered in chemical interactions. Let’s consider an example of how they interact.
The Ideal Gas Law
The ideal gas law utilises a variety of observable gas properties to create one universal equation to model ideal gas behaviour. It is derived from the following laws:
- Boyle’s Law: pressure and volume are inversely related at constant temperature and moles of gas.
- Charles’s Law: volume and temperature are directly related at constant pressure and moles of gas.
- Guy-Lussac’s Law: pressure and temperature are directly related at constant volume and moles of gas.
- Avogadro’s Law: volume and moles are directly related at constant pressure and temperature.
As a result, a ‘gas constant’ can be produced by combining these laws together. Therefore:
[latex]\dfrac{PV}{nT}=\text{constant}=R[/latex]
which is usually rearranged as follows:
[latex]PV=nRT[/latex]
This equation is called the ideal gas law. It relates the four independent properties of a gas at any time. The constant R is called the ideal gas law constant. Its value depends on the units used to express pressure and volume (see table 5.5.1).
Numerical Value | Units |
---|---|
0.08205 | [latex]\dfrac{\text{L}\cdot \text{atm}}{\text{mol}\cdot \text{K}}[/latex] |
62.36 | [latex]\dfrac{\text{L}\cdot \text{torr}}{\text{mol}\cdot \text{K}}=\dfrac{\text{L}\cdot\text{mmHg}}{\text{mol}\cdot \text{K}}[/latex] |
8.314 | [latex]\dfrac{\text{J}}{\text{mol}\cdot \text{K}}[/latex] |
This idealised law is not perfectly representative of real gases, as it does not take into account the intermolecular forces experienced by gas molecules. Instead, an alternative formula known as the van der Waals equation is utilised to predict real behaviours. However, the idealised equation allows us to observe general trends.
Let us imagine an experiment conducted measuring the ambient pressure of an ideal gas in a sealed container at room temperature. On a cold night, a faulty laboratory in Antarctica that is still waiting for the heating to be repaired reports minimal amounts of pressure experienced by this gas at room temperature. They note that their measurements fluctuated greatly over the night and publish their findings in a well-respected journal. These findings did seem to contradict established gas laws; it would be a shock for the entire science world! With controversial results, a laboratory in Australia, more well equipped to maintain a constant room temperature of 25°C, performs the same experiment and invalidates their results, noting that Guy-Lussac’s findings and the ideal gas law still stand. They recommend an available engineer to assist the Antarctic researchers in repairing the air-conditioning! It would seem that the environmental conditions in which a reaction occurs are of utmost importance.
Standard Laboratory Conditions
It is not just gas molecules that are influenced by laboratory conditions. Within chemistry, heat and pressure influence all reactions to some degree. As the reproducibility of experiments is needed to validate any findings, scientists must take note in their writings and methodologies of factors such as temperature, pressure, humidity, or any other external factor which could alter the outcome of an experiment. Often, experiments are conducted without any change to these factors.
As such, the term standard laboratory conditions describes a typical, room-temperature laboratory. Standard laboratory conditions (SLC) are centred around 25°C (298.15 K) at 1 atm (101.325 KPa), experiencing 1g (9.81ms-2) of gravity. The reason for this vagueness in the definition is due to geographical differences between laboratories. Aspects such as height from sea level (influencing pressure and temperature) and distance from the poles (due to Earth’s imperfect shape presenting differences in gravity) will cause laboratories to experience differences in conditions. Generally, the differences between laboratories (that are well maintained) are so minor that they are negligible in standard experimentation. However, for work and research desiring extreme accuracy, these conditions can have notable impacts on outcomes.
Key Takeaways
- The ideal gas law describes relationships between P, V, T and n of a gas whose component molecules are assumed to have NO intermolecular interactions with each other (and not directly to do with ‘ideal conditions’).
- Most experiments are completed under standard laboratory conditions, with no extreme deviation from average conditions.
Exercises